Deep Dive 6
Why is a Network Analysis of the Petrochemical Industry useful?
1 HancockHamlin ltd.,
No Address given.
Lorem ipsum dolor sit amet, consectetur adipiscing elit. Suspendisse varius enim in eros elementum tristique. Duis cursus, mi quis viverra ornare, eros dolor interdum nulla, ut commodo diam libero vitae erat. Aenean faucibus nibh et justo cursus id rutrum lorem imperdiet. Nunc ut sem vitae risus tristique posuere.
A network analysis of the petrochemical industry reveals the complexity and interconnectedness of the sector and indicates how changes made in one plant or location would have implications elsewhere. This knowledge helps policymakers to target strategic locations or companies for emissions mitigation.
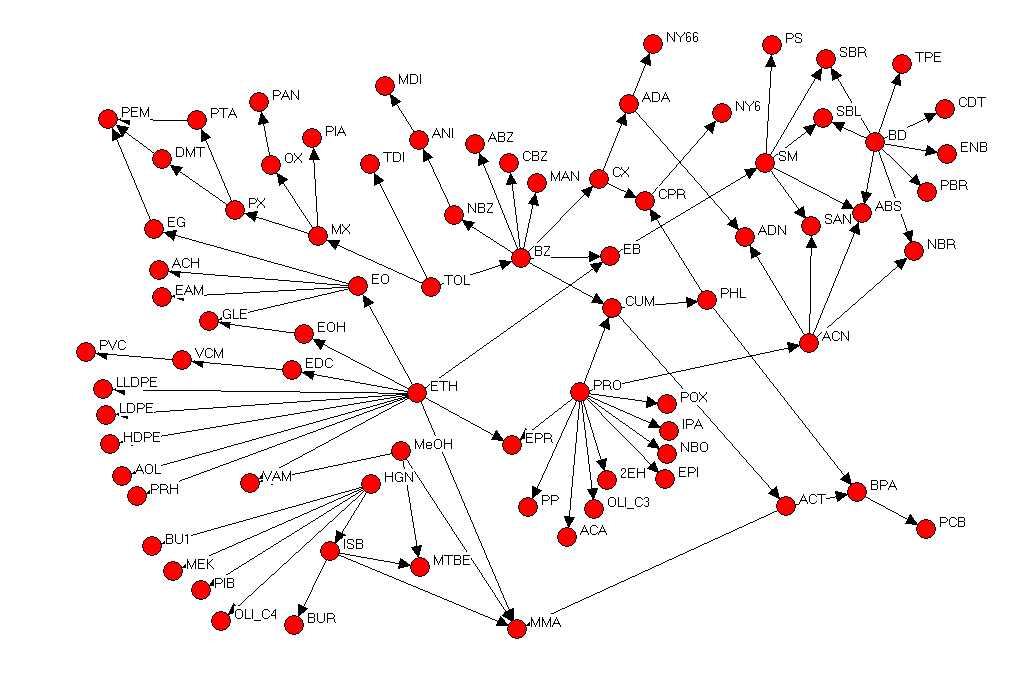
Figure 1 Generic Production Map for the Western European Petrochemicals Industry (2005)
the problem
In contrast to many manufacturing industries, the diversity of products in the petrochemical sector increases as you move further down the value chain, and outputs from one plant are often feedstocks for another. This complexity makes the chemical industry even harder to decarbonise than other sectors, as there is no single solution that can be applied across the whole industry. Policymakers and influential actors in the petrochemical sector need a better understanding of its structures, networks, and interdependencies if emissions mitigation efforts are to succeed.
Methods
Building on our previous study focused on Western Europe, we use a network analysis to explore the different ways in which places and actors in the sector are connected in networks of interdependence. This provides insight into who has influence in the industry and where stakeholders could target their limited resources to have maximum impact on carbon emissions. We select three key layers of analysis: products, location, and ownership.
Key Results
Products
- Network maps of the chemical production value chain show its interconnectedness, with many products feeding into the production of other chemicals.
- Product maps can be produced for individual companies to reveal where in the value chain a company operates, e.g. whether they focus more on downstream chemicals or bulk commodity chemicals. This has potential implications for circularity.
Location
- Petrochemical plants located near one another often share core utilities, use chemicals or by-products from one another, and exchange skills and knowledge. This can either be used to drive positive change or become a major obstacle.
Ownership
- The industry has a complex range of ownership arrangements. Applying social network analysis allows us to identify which industrial actors have the most influence to either help or hinder policy and change.
The insights from this multi-layered network analysis can help policymakers and industry actors to effectively target and tailor their different mitigation approaches for the relevant locations, products, or companies.
Keep scrolling to read the full Deep Dive
Explore more Deep Dives
Deep Dive 1
Model-based energy and emission analysis of ammonia production for improved process sustainability
Ammonia production is highly unsustainable. Our model-based analysis reveals that in the US, emissions from the ammonia industry could be reduced by 10% by taking measures to enhance the efficiency of existing plants. To reduce emissions to near-zero by 2050, more fundamental changes to production pathways are necessary. Low-carbon technologies such as water electrolysis and carbon capture and storage will need to be developed and deployed to achieve this near-zero target with minimum cost.
Deep Dive 2
Global mass flow of nitrogen fertilisers and mitigation options
Nitrogen fertilisers account for 5% of global greenhouse gas (GHG) emissions. Our global mass flow model uses a whole-systems approach to explore combinations of mitigation interventions. If all the interventions we consider were applied together, GHG emissions from synthetic fertilisers could be reduced by 84% by 2050. The single most effective intervention is increasing the efficiency of nitrogen fertiliser application to plants during their use phase.
Deep Dive 3
Recycled polymers as a feedstock for chemical manufacturing supply chains in the United States: A network analysis for polyethylene pyrolysis
If 5 Mt of PE waste were chemically recycled each year, and the resulting ethylene and propylene were put to use by the chemical industry, other sources of these chemicals would be displaced. The knock-on effects of this would span a large number of products and processes. These wider repercussions need to be considered in any evaluation of chemical recycling as an emissions-reduction strategy.
Deep Dive 5
The economic context
We need to consider the potential wider economic impacts of climate change mitigation actions taken within the petrochemical sector. Therefore, we have developed an integrated macroeconomic model that combines both material and economic flows. The model is calibrated for the US context so that it represents the real-world stocks and flows of mass, energy, population, and money.
Deep Dive 6
Why is a Network Analysis of the Petrochemical Industry useful?
A network analysis of the petrochemical industry reveals the complexity and interconnectedness of the sector and indicates how changes made in one plant or location would have implications elsewhere. This knowledge helps policymakers to target strategic locations or companies for emissions mitigation.
Read the full Deep Dive
Abstract
The petrochemical industry is complex, heterogeneous, and interconnected. In contrast to many manufacturing industries, the diversity of products increases as you move further down the value chain and outputs from one plant are often feedstocks for another.
This complexity makes it even harder to decarbonise than other sectors as there is no single solution that can be applied across the whole industry, yet those unfamiliar with the sector often strive to do so. Therefore, there is a need to improve our understanding of the structures, networks, and interdependencies of the petrochemicals sector among policymakers and influencers.
The Geographical Archetypes of the Petrochemicals (GAP) model developed through C-THRU begins this process by proposing eight archetypes that represent a simplification of the industry. The archetypes are extremes which show how different markets occupy different positions within the petrochemicals sector depending on three interconnected factors: the supply of hydrocarbons, the demand for petrochemicals from manufacturing, and the economic freedom of the state. The model shows how varied the industry is and hypothesises the types of network structures and characteristics that would be anticipated in each of these archetypal extremes. This can help guide and tailor interventions that will be more impactful in a particular location and that have been anticipated at the country level.
The GAP model is just a first step, however, with the focus now turning towards validating the hypothesised network structures and characteristics of each archetype by conducting a network analysis of the global petrochemicals sector. The expectation is that this work will confirm which different types of networks of actors operate in each archetype. This knowledge can help inform policymakers and companies of how best to facilitate change at a local level to drive global change and impact.
But this raises two questions – what exactly is network analysis? Why will policymakers and others find this approach useful?
Scroll to keep reading
Introduction
What is Network Analysis?
Network analysis has typically been used to understand social structures. Applying tools and techniques associated with networks and graph theory, the strong and weak ties within a social grouping can be established. In addition, inference can be made from the network structure as to whether it self-organises or has some externally imposed hierarchies. From a social network perspective, this enables the identification of key influencers and change agents who can propagate information through these centrally networked actors.
Taking a social network analysis approach to an industry sector enables the links within the industry to be identified and assessed to determine whether the sector displays similar characteristics. In 2005 we used these tools in a previous study to investigate the networks of the petrochemical industry in Western Europe and concluded that the sector did exhibit characteristics and structures typically seen in self-organising social networks.1 This helped explain how certain companies had flourished in the industry whilst others struggled. Although the research was constrained to one region, it is anticipated that a global analysis will further validate the use of social network analysis techniques in industry and identify different network structures that will align with the GAP model expectations.
The research into Western Europe can be used to illustrate the application of network analysis tools. A key output of the work was a series of network maps focusing on the products, locations, or owners of the petrochemical industry in the region at that time.
Scroll to keep reading
Method
Products
The first network map shows a typical chemicals production value chain. Whilst some chemicals are used on their own, many are combined with other chemicals to create new chemicals. The basic map is applicable across the whole petrochemical sector, though production locations of the different components will vary considerably.
Figure 1 shows the product network map created for the Western Europe research. There are many interconnections, as shown by considering ethylene (blue dot) and propylene (green dot), which both feed into the production of many other chemicals.
This product map was used to infer links between chemical plants. For example, if Ethylene Oxide (EO) is a precursor for Ethylene Glycol (EG) and there are plants that produce each of them situated in the same location, these are likely to be connected in some way that may not be apparent by looking purely at the ownership of the plants.
There are limitations to this simplified product map, some of which may be addressed in the planned global network analysis. Firstly, the core petrochemical feedstocks are not incorporated, so the value chain does not trace back to the hydrocarbon sources. Secondly, it does not account for the by-products that are created alongside the core chemicals. Other chapters in this report present product-specific maps of material flows that do incorporate upstream sources and by-products. Learnings from these will be used to improve the approach in the industry network analysis work, but the simple product map shows basic chemical links and adds granularity to the detail of location and ownership analysis.
For example, it enables an individual product map to be produced for a company, as in Figure 2, which shows the map for what was then Dow. This type of analysis can be used to understand where in the production value chain a company operates – i.e. whether they focus more on downstream chemicals or bulk commodity chemicals, etc.
Locations
The second focus of network analysis in our original research was location. A petrochemical plant was considered the lowest unit of analysis from a geographical perspective. A plant may produce just one chemical, but often comprises several different product streams manufacturing multiple chemicals either by design or as by-products. It is common for a plant to operate near other chemical plants or co-locate with a number of plants to form a petrochemical site. The plants often share core utilities and the chemicals or by-products from one are often utilised as feedstock in another chemical process.
In the Western European petrochemical research, in addition to the formal links between plants established by product flows, it was felt that there was likely to be a skills and knowledge exchange based on the people in that area. Therefore, to capture this wider interchange of people and materials, a proximity boundary of 75km was set based on the premise that this represented a maximum travel time of one hour. Plants operating within a radius of 75km were therefore considered co-located and this generated a series of additional locations all assigned the prefix ‘Greater’.
This resulted in network maps such as Figure 3 which shows the production chain map for Dow fragmented into product streams and locations. This gives two main sites with extensive product value chains, and five smaller sites producing only a small number of chemicals.
Ownership
The final layer of analysis is that of ownership. The petrochemical industry has a multitude of joint ventures and co-ownership arrangements, often a direct result of the interconnectedness of the products and feedstocks. Unravelling the complexity of who owns which plant is no easy task, with many companies operating through multiple subsidiaries. This generates a network map of very dense connections, as seen in Figure 4 which shows the companies linked by location, and Figure 5 which shows locations linked by common operators.
Applying social network analysis tools to these different maps provides insight into the interconnectedness of the industry. Twenty years ago, computing capabilities were limited and only allowed for analysis of either geography or ownership, but technological developments now enable greater depth of interrogation. It is anticipated that revisiting the original analysis and updating and extending it with current data will enable a global network map of the petrochemical industry to be created.
Scroll to keep reading
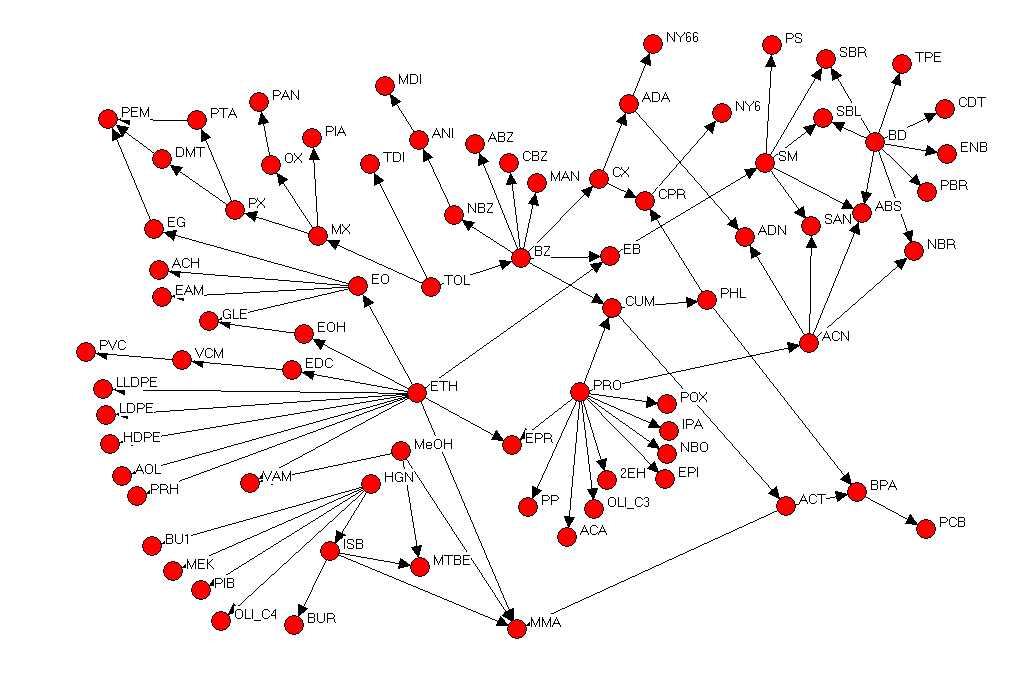
Fig. 1 Generic Production Map for the Western European Petrochemicals Industry (2005)
.png)
Figure 2 Production Chain Map for Dow (2005)
.png)
Figure 3 Production Fragment Map for Dow (2005)
.png)
.png)
Fig. 4 Network of Companies Linked by Common Operating Location (2005)
Fig. 5 Network of Locations Linked by Common Operating Companies (2005)
Results & Discussion
Why is it useful?
As already indicated, the petrochemicals sector is interconnected and hard to decarbonise given that it is dominated by the use of hydrocarbons as a source material. However, with better knowledge and understanding of the industry’s structure, characteristics, drivers, and constraints, mitigation efforts and policies can be targeted more effectively.
One of the key outputs of the network analysis is an understanding of influencers within the sector. By examining the connections between companies and their degrees of interconnectedness it is possible to identify the most influential actors within that network. The same is true of locations, with connections between sites showing how dense the operations are. This again shows the concentration of influence. These key operators and locations become critical instigators or barriers to change. A positive action taken by one of these influential nodes can have a cascade effect on their network, but the reverse can also be true.
Table 1 from the original research shows how many companies could be reached across Western Europe by interacting at that time with Dow: a staggering 61 companies in total. This figure jumped to 80 if TotalFinaElf was included.
This suggests that if you only had resources to influence one company then Dow was the most relevant at that time.
The picture becomes even more interesting when considering locations. As Table 2 shows, if there were only enough time or resources to focus on one location then Greater Antwerp would have reached 76 companies. However, if there were the opportunity to work with two locations, then selecting Greater Bilbao and Greater Oberhausen instead would reach a greater number of companies.
This insight can help policymakers and investors understand how individual actors or geographical locations can help or hinder change and which are the most critical. This is a particularly relevant factor when having to prioritise finite or scarce resources.
Mapping the interconnectedness of the industry highlights where technological interventions might be most impactful. A solution to decarbonise one specific chemical process might be effective in isolated plants, but in a highly connected site, the impact further down the value chain could undermine any emissions reductions in that initial plant. This is also relevant for circularity and recycling efforts. Interconnected and co-located plants are typically optimised for efficiency and waste minimisation, but if appropriate quality recycled materials could be used at the start of the production chain this could then generate a green cascade through other chemicals.
The original 2005 research1 also revealed the impact of major chemical pipelines on the industry. The principal ethylene pipeline in Europe operated by ARG runs 495km linking Cologne and Ruhr to Antwerp with at least 18 major chemical plants along its path. Incorporating pipelines into the Network Analysis is important as previously disparate sites are then recognised as part of a more significant influential cluster. The presence of pipelines is likely to be relevant when instigating change; a pipeline that essentially has multiple companies feeding into it and multiple companies extracting from it could be used as a catalyst to increase the use of recycled materials or could become a block. A pyrolysis plant that can recycle materials back to ethylene that is chemically identical to virgin ethylene could feed into the pipeline, kick-starting a circular economy approach across a large geographical area and increasing the production of chemicals with recycled content downstream. Similarly, pipelines within a closed system of sites can help facilitate circularity if the operator and participants work together.
Therefore, the additional insight that the network analysis can provide is invaluable in helping policymakers and industry actors tailor and target their different decarbonisation and mitigation approaches to be most effective, whether that is in a particular location, with specific companies, or focusing on an individual chemical or product value chain.
Only by adopting different approaches that are relevant to that geography, sub-sector, or production process will decarbonisation targets be achieved.
Scroll to keep reading
Table 1 Company Networks – most critical nodes (2005)
Table 2 Location Networks – most critical nodes (2005)
references
- Hamlin, C. (2005) Distributed knowledge and intelligence in the extended enterprise: A network analysis in Western Europe. For the Advanced International Summer School ‘Innovation in the Extended Enterprise’, Italy, 2006
Explore more Deep Dives
Download this Deep Dive
Lorem ipsum dolor sit amet, consectetur adipiscing elit. Suspendisse varius enim in eros elementum tristique. Duis cursus, mi quis viverra ornare, eros dolor interdum nulla, ut commodo diam libero vitae erat.